Sedimentary isostasy
Isostasy is a model that describes how upper lithospheric buoyancy adjusts to any significant change in overburden pressure; it responds to significant additional masses of ice or sediment by subsiding. This equilibrium buoyancy model is supported by a hypothetical mobile magma that exists at depths not yet penetrated by the drilling rig.
Sedimentary isostasy is a related model that addresses how space is created below base level to accommodate additional influxes of sediment. This model assumes that relative sea level remained stable for extensive periods of geological time, despite the continental crust's level changing intermittently throughout the Phanerozoic. The significance of the model is that it can help explain complex geological events observed in sedimentary basins across the globe. In basin analysis it provides a viable alternative to the Sequence stratigraphy model, which implies that sea levels frequently rose above present sea-level and that the crust independently oscillated up and down throughout geological time. It also provides firm theoretical support for the emerging precepts of tectonostratigraphy.
History of the sedimentary isostasy model[edit]

The origin of the sedimentary isostasy model is secured in a letter from John Herschel to Charles Lyell dated 20 February 1836. Herschel was writing from Cape Town, where he was engaged in an astronomical survey of the southern skies. In this letter he discusses the relationship between crustal uplift and submergence using the limited technical vocabulary of his time. The accompanying sketch shows his firm grasp of a viable mechanism to explain the balance between sediment deposition and adjacent tectonic uplift. Unfortunately his insight only achieved publication two years later in Paragraph I of an Appendix to Charles Babbage's broadly philosophical Ninth Bridgewater Treatise[1] Herschel was a polymath; his fundamental contribution to geology was smothered by his immense contributions in other fields and shortly was forgotten in the heat of geological discovery during that decade.

Charles Babbage (1791–1871) and Charles Lyell (1797–1875) had already been considering possible relationships between granite and stratified rocks. Babbage applied his Analytical engine to the problem of granite compressibility. In 1837 Lyell inserted a frontispiece in the 5th edition of his Principles of Geology that marks a major advance in deep crustal interpretation.[2] Following Herschel's model, it shows a layer at no great depth, where the combination of heat and pressure renders rocks pliable; at greater depths still, they become completely degraded to a mobile granite melt. The lateral flow of melt implied by that diagram provided compelling support for Herschel's concept of sedimentary isostasy.
Homeward bound from his voyage around the world aboard HMS Beagle 1831–1836, Charles Darwin (1809–1882) HMS Beagle called at Cape Town on 3 June 1836 and visited Herschel accompanied by Captain FitzRoy. On return to England, Darwin reported some key evidence observed along the shores of Patagonia

- 'Considering the enormous power of storm waves, it is unlikely that a sedimentary deposit could have survived the ordeal of beach erosion, unless before uplift, it had considerable thickness and a wide extent offshore'. If it were uplifted slowly, the coast should have faced the present ocean with a gentle slope, rather than by a series of steps. This realisation led Darwin to expand the description of sedimentary isostasy: 'Also, on a moderately shallow bottom, where most living marine creatures are found, it seems impossible that a thick expanse of sediment could be deposited widely, unless its bottom sank sufficiently to accommodate successive layers. I am inclined to believe these subsiding movements were accompanied elsewhere by those of elevation'.[3]
Amanz Gressly (1814–1865) studied strata in the Swiss Jura Mountains and described a vertical succession of groups or terrains there. He then traced beds in horizontal dimensions and encountered marked lateral changes in lithology and paleontology in each stratum. Addressing these lateral changes, he proposed (1838) the term facies and identified sandy, muddy, littoral pelagic and other facies, describing them in terms of both sedimentary type and their inferred environment of deposition.[4]
The next significant step was made by the Russian geologist Nikolai A. Golovkinsky (1834–1897). He formulated his Reverse rule, which states that the boundaries of lithologically and palaeontologically similar layers regularly slide in time when traced laterally.[5] This rule substantiated the primary asynchrony of implied biostratigraphic boundaries. Thus, age-related disposition of the boundaries was associated not only with facies differences in same-aged layers, but also with the palaeobiogeographic features of their faunas. Golovkinsky realized that this contradicted "conventional" views about the process of sedimentation, concluding:
the common belief in the sequence of formation of successive layers is not true.
(Golovkinsky, 1868, p. 125) and remarked that that this would seem paradoxical to many geologists.
In 1894 Johannes Walther (1860–1937) enunciated the Law of Correlation of Facies,[6] which in essence states that within a single sedimentary cycle, the same succession of facies observed in a vertical succession is also present laterally. This fundamental principle[7] traces its origin from the earlier studies of Gressley and Golovkinsky, and Walther's Law remains a guiding principle of European stratigraphy.
In 1905 Grabeau (1870–1946) described the key concept of disconformity and ideas of stratigraphic onlap and offlap.[8] He was much influenced by the works of Walther and he describes the outcome of Walther's Law in a subsiding sedimentary basin: it will be seen that no two portions of the bed along a line transverse to the seashore will be of the same age, each sea-ward portion will be younger than that lying next to it nearer the land. Thus the formation line, limiting the basal conglomerate or sandstone, will run diagonally upward through the planes of synchronous deposition. However, when illustrating a marine transgression event p. 726 and Fig. 144 he failed to appreciate that Walther's Law would not apply if sea level were rising slowly to form an inverted facies sequence:

... land-ward migration of the coarser deposits under uniform conditions (implies) that the changes in any given bed, from the shore sea-ward, will be exactly duplicated by the changes in a given vertical section from the base upward ... the coarse bed deposited directly upon the old sea-floor of crystalline rock is succeeded upward by a somewhat finer bed, since coarse material has, by continuous subsidence, migrated further landward. Grabeau's transgressive model cannot form a valid basis for cyclic sequences because each subsequent crustal uplift above sea level would cause erosion of the previous layer.
Grabeau's regressive model (p. 733 and Fig. 148), by contrast, anticipates many characteristics of the Chronosome model:

... For a stationary sea-level, regression of the seashore will take place ... the shore zone would creep out over the deeper water deposits, the transition from one to the other being rather more abrupt than in the case of a slowly subsiding sea-floor. These relationships are shown in Fig. 148, where it will be noted that the diagonal rise of the shore-formed stratum is from the shore sea-ward, whereas in transgressive movements the shore-formed stratum or basal bed rises diagonally land-ward. Such effects are seen in basins (of the continental interior), where the upper beds extend farther toward the mountains from which the material has been derived.
Advances in stratigraphic understanding stagnated after the 1914–18 World War, whilst debate continued about the relative roles of sea level and crustal subsidence. Earth scientists turned to Milankovich theory, meteorite impacts and other catastrophism theories to explain periodic events. During that fallow period for intra-continental stratigraphy, the role of isostasy in crustal movements received a boost from studies of the elevation of Scandinavia during the Holocene, which then was thought to be a response to melting of a thick Pleistocene ice sheet. The removal of that mass postulated by Swedish geologists gave fresh impetus to the theory of isostasy, but any complementary sedimentary loading of North Sea basement was not considered by their investigations.
In 1917, Joseph Barrell was one of the first to discuss composite rhythms of deposition within the context of what is preserved in the stratigraphic record. His concept of a continuously variable base level laid precarious foundations for analytical and seismic stratigraphy, and this approach won eventual endorsement by Exxon's Sequence stratigraphy model that arrived 60 years later, although that explanation is still controversial.
The chronosome model[edit]
Thick and extensive sedimentary basins develop below a stable sea level as a result of the deposition and accretion of a succession of sedimentary Chronosomes. A Chronosome is a sedimentary rock unit bounded by planes, each defined by the time of simultaneous deposition of various lithologies.[9] Schultz (1982) proposed the term Chronosome for the 3D space bounded by time planes whilst acknowledging that that seismic reflection horizons do not correspond with time planes.[10] Griffiths and Nordlund (1993) recognized the value of the chronosome term but considered that lithosomes had greater relevance to seismic correlation, since reflection bounded units are much thicker than the typical chronosome. They considered a chronosome (upper) surface "the nearest we can approach to a paleotopography. The time duration of a chronosome will vary according to the resolution of the observational technique, but at the limit of resolution the chronosome will always provide the optimum predictive unit." [11] The concept had its origins in 1958 following the publication of Time Stratigraphy by Harry E Wheeler (1909–1989).[12] Wheeler diagrams are cross-sections that show the geographic location and order in which chronosomes, bounded above and below by discontinuities were deposited.[13] Wheeler charts show the location and lateral facies gradation of strata and the sequence of deposition in an arbitrary brief time interval. Current examples do not depict the thickness or vertical facies gradation between successive units, so they do not enhance understanding of cyclic deposition.
The problem addressed by the chronosome model is how to account for repeated groups of strata, each group having a dominant component containing fossils of the upper neritic zone that imply marine recession, yet collectively the cyclic sequence has accumulated to vast thicknesses well below sea level. So, whilst sea level appears to be retreating, paradoxically and periodically it returns to exactly the same base level.

Then in a quasi-organic process, it reproduces the same facies sequence with a similar thickness to its predecessor.
In an outcrop or well boring, a bed of sand resting on silt and mudstone taken together forms a distinctive clastic chronosome sequence. Each vertical sequence of facies is closely related to the downslope lateral sequence of facies. Thus, at one instant in time, sands are deposited close to the shore, silts and shales form the seabed farther offshore, illustrating the multidimensional facies relationship first recognized in the Jura Mountains by Gressley, 1838. Over millennia, as sand facies accumulates seawards, previously distal facies get buried and the coastline advances on top of the silt and mudstone. This relationship between vertical and horizontal facies follows Walther's Law. Surfaces that portray levels of simultaneous sediment deposition curve upwards from the horizontal, where vertical accretion is slow in deeper water and become steeper in shallow water where the beach front progrades towards offshore. A similar process operates in carbonate basins, where sabkha facies above the shoreline is followed seawards by bioclastic carbonate sand in shallow water, grading to a lime mud facies farther offshore. Here the driver of the system is the growth and accumulation of a bioclastic facies that grows organically, accumulates in a narrow coastal beach, and gradually extends offshore across the shelf until the single chronosome addition to basin mass exceeds a trigger point that initiates an apparently rapid transgression.
Episodes of subsidence allow the entire basin to accommodate each repetition of chronosome deposition; these explain the accumulation space paradox outlined above. Thus, after the crust subsides rapidly by say 50 to 100 metres, marine water floods across the most recent chronosome, a new coastline is established farther up the sloping craton and seaward regression begins again.

On the deeper shelf each chronosome would comprise thinner distal facies and the distal seabed slope would become proportionately steeper.
Cyclothems or chronosomes?[edit]
The cyclothem term is an inexact equivalent of a chronosome model that forms a single regressive sequence. However the cyclothem term has led to much confusion through mis-application in Sequence stratigraphy. It often leads to consistent though invalid boundary planes in its definition of a regressive cycle and perhaps should be regarded as deprecated or obsolete. A thorough historical review of the role of cyclothems was made in 1964 by Marvin Weller for Kansas Geological Survey:[14] This makes it clear that boundaries chosen to define cyclothems were often inconsistent ad hoc choices that lack the constraints of an overall depositional, geoid and diastrophic model.
Stratigraphic boundaries should respect both biostratigraphy and facies analysis, which require detailed regional knowledge. This is beyond the scope of seismic stratigraphy and remains difficult even in cored wells. Consequently, notional cyclothem transgressive boundaries have often been chosen at the wrong 'turning point' in a repeating sequence of facies. In addition, unsubstantiated attempts have been made to explain cyclothems by reference to Milankovich cycles or repetitive climate changes. In recent years many stratigraphers prefer an Allostratigraphic model approach, which remains receptive to possible changes in relative sea level. Allostratigraphy is based on the sub-surface correlation of chronstratigraphically significant surfaces through rocks of varying lithologies. It is a way of defining and naming discontinuity bounded rock successions, emphasising unit mappability rather than origin in the context of sea level change. At present, a quasi-uniformitarian approach to stratigraphy using the chronosome model is preferable, pending better definition of geoid changes.
Correlation across a stable basin[edit]
In a relatively stable carbonate basin such as the Persian Gulf, scores of stacked chronosomes can be correlated, often bed by bed across the region. Their ages range from the Jurassic Arab Dharb Formation through to the Miocene. Successive chronosomes stack up to four kilometres in thickness, becoming thinner over rising salt domes. Regionally and collectively the Mesozoic and Tertiary succession thins towards the Arabian Craton and thickens towards Iraq and the Zagros Mountains. Repeated small basin floor subsidences provide accommodation space for the initiation and spread of their respective chronosome increments. In areas of more complex structure, individual chronosomes can be correlated within specific fault blocks and otherwise can assist restoration of folds and faults to their depositional forms. A significant characteristic of the Persian Gulf sequence is the prolific development of cyclic sedimentation. The causes of widespread cyclothem occurrence are still disputed by stratigraphers but the implications of the Sedimentary Isostasy model may lead to a better understanding of this issue.
Chronosome sea level model[edit]
There is no stratigraphic or geomorphological evidence that provides unequivocal support to a sea level significantly higher than the current maximum tidal range. Any apparent exceptions to this rule can be explained otherwise by epeirogenic or orogenic crustal uplifts or by local gravity anomalies that have disturbed the present geoid model. Chronosome theory requires that sea level was fixed relative to a geoid with an equatorial diameter that remained stable and constant with only occasional catastrophic interruptions during the Phanerozoic Era. The chronosome model acknowledges that the Earth's geoid was disturbed intermittently by plate tectonics and meteorite impacts throughout that period. Events such as the global Maastrichtian Transgression[15] established a new and stable base level that defined a new geoid based on a revised Earth's equatorial diameter. Tectonic activity related to such catastrophic disturbances caused disconformities that interrupt the chronosome model, which resumed its cyclical progress when the geoid attained equilibrium.
Chronosome isostasy model[edit]
At a certain point in time the basin-wide thickness and mass of a single chronosome exceeds a nominal isostatic threshold; the entire basin sinks simultaneously, sea water sweeps across the previously deposited chronosome sand layer and a new coastline is established farther up the craton slope, causing the recessive deposition process to begin again. This isostatic model assumes that mass per unit area is evenly distributed across most of the basin because the heavier and thicker proximal facies gradually spreads across most of the basin. However Bailey Willis pointed out that: Each region has experienced an individual history of diastrophism, in which the law of periodicity is expressed in cycles of movement and quiescence peculiar to that region.[16] It follows that global correlation using Sequence Stratigraphy may not be reliable, except for very broad intervals of time.
Cyclicity mechanism underpinning[edit]
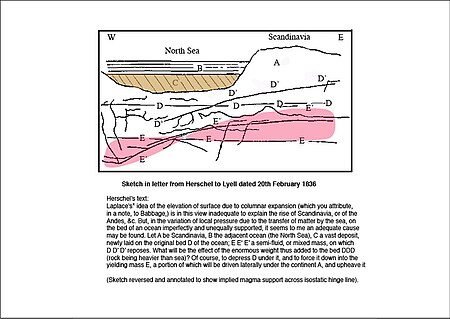
The structure of the Viking Graben and adjacent areas was established from seismic and well data during the late 20th century.[17]
Its relationship with the Scandian high level peneplain was established only in 2018 when Japsen et al. described evidence on the Hardangervidda Mountains in southern Norway that helped validate Herschel's model.[18] Herschel's letter apparently influenced Charles Lyell's Principles of Geology Book frontispiece (5th edition 1837). This shows rocktype sequences in a stable sedimentary basin. Below about 3,000 metres of porous water- and hydrocarbon-saturated sedimentary rocks are a further 2,000 metres of sediment with porosity mostly filled by diagenetic cement. Beyond that level, sediments are metamorphosed by temperature and pressure to form quartzites, schists and marbles with ground water absorbed as crystal components. Deeper still, rocks reach a stage of ultrametamorphism into gneiss and migmatite, a contorted metamorphic rock containing a granitic partings. [19] Finally,

the combination of temperature and pressure exceeds the phase transition threshold of supercritical water,gas [20] [21] which assists in the production of an extremely mobile granitic melt(granulite) at a depth of 10 to 15 kilometres. [22] The current chronosome model assumes that the thickness of this fluid zone is limited by a very precise combination of physical and chemical factors to form a thin layer, implied by the amount of intermittent subsidence to be less than 100m. thick. This zone likely coincides with the Conrad discontinuity[23]. Above that level, any reduction in temperature and pressure causes water to drop below its supercritical phase threshold and minerals in the granulite crystallize in sequence. This occurs not far above the Conrad level because granulite rarely reaches the surface as rhyolite lava; if it were to reach the surface, it could cause a catastrophic eruption.[24]
At the Conrad Discontinuity migmatite encounters a process of anatexis and differentiation, the melting and gravity separation of the basic heavy minerals, which sink to form a restite of immiscible basic magma. The separate granulite residue has its mobility further enhanced as a consequence, and overburden pressure causes it to seek a lateral passage away from the centre of the basin. As the granulite's initial volume is expelled laterally, the entire basin sinks into the space vacated by magma, thus placing the next level of transitional migmatite directly on top of the restite, where it begins to melt and perpetuate cyclical processes throughout the basin. There is usually an ancient craton on at least one side of an extensive sedimentary basin. When the lateral impetus of granite magma reaches a craton boundary it splits the craton horizontally at Conrad level and raises or tilts a fault block upwards to form a new horst feature. Successive magma injections beneath it raise the horst block further by stages and related uplift and erosion of the block contribute a further influx of sediment to form a new chronosome in the basin.
Practical applications of the chronosome model[edit]
Chronosomes are the most productive environments for the growth of plant and animal life and formed source rocks for petroleum in both terrigenous and carbonate buried sediments.[25][26] Extensive plains that extend behind the coastline were a favourable environment for prolific plant life that became buried quickly by each marine transgression. In the Upper Pennsylvanian, Permian and Cretaceous coal and lignite were sources for waxes and resins that produce asphalt and methane under temperature and pressure conditions of deep burial. Rivers carried decaying vegetable humus into an adjacent basin where it became buried in association with silts and muds. The intertidal zone is a prolific habitat for seashells, crustaceans, bird droppings and sea grass. Below wave base on temperate, terrigenous shelves, kelp forests restrict the offshore flow of sediments. This increases the rate of sediment deposition and their buried remains further contribute to waxy source rocks. Blue and green marine algae, diatoms and lithothamnium also offer promising source material for petroleum in both temperate terrigenous and tropical carbonate basins. On carbonate platforms the coastal plain is a predominantly sterile sabkha environment. Reef-building corals seashells and crustaceans accumulate on its shallower neritic zones, whilst foraminifera, sponges and algae get buried beneath a rain of carbonate mud in deeper water. Clearly, the productivity of organic life greatly assists gravity, insolation and climate in advancing the accumulation of successive chronosomes throughout the world.
Fluid flow in a petroleum reservoir is influenced by the distribution of porosity and permeability in its component strata. The identification of 'flow units' within this sequence can help determine appropriate layers for use in reservoir modelling. These models are based on a three-dimensional grid of cells. The flow characteristics and fluid contents of these cells determine the outcome of a computer program that simulates the effects of reducing and increasing pressure in various parts of the reservoir. This reservoir model is based on modelled geometry of facies, established from cores in early development wells. Thus its stratigraphic parameters are based on layers that combine a Chronosome model with a Wheeler Diagram. Practical limitations on cell size ensures that initial models provide only a provisional, yet indispensable likeness of facies distribution. [27][28]
Comparison of the sedimentary isostasy model with the sequence stratigraphy model[edit]
The fundamental difference between these models is their contending views about the role of eustatic sea level. Despite early acceptance of the Sequence stratigraphy paradigm in academia and industry, many experienced geologists still retained cogent doubts about its wider validity. In 2010 Andrew Miall made a forensic analysis of the attributes of the Sequence stratigraphy model. He concluded in that its basic premises have never been proved.[29] Early objections to sequence stratigraphy were that a synchronous regional or global signal cannot be generated where relative sea level responds to two or more processes and that it subordinates conventional chronostratigraphic methods to a hypothetical global cycle chart.[30] Viability of the rapidly changing relative sea level model envisaged by sequence stratigraphy was debated by Hallam, Vail and other experienced geologists in Eustasy, a book edited by R.H. Dott Jr., 1992.[31]
References[edit]
- ↑ Babbage, Charles (1838). Ninth Bridgewater Treatise Appendix. London: John Murray. Search this book on
- ↑ Lyell, Charles (1837). Principles of Geology. London: John Murray. Search this book on
- ↑ Darwin, Charles R. (1860). Journal of researches into the natural history and geology of the countries visited during the voyage of H.M.S. Beagle round the world, under the command of Capt. Fitz Roy R.N. London. London: John Murray. Search this book on
- ↑ Gressley, Amanz (1838). "Observations geologiques sur le Jura Soteurois". Nouv. Mem. Soc. Helvet. Sci. Nat. 6: 1–112.
- ↑ Golovkinsky, Nicolai A (1868). "Formations primaires de la partie centrale du bassin Kama-Volga". Matér. Géol. Russie, 1868: 1–112.
- ↑ Walther, Johannes (1894). Einleitung in die Geologie als historische Wissenschaft. Jena: G. Fischer, Bd. 3. pp. 535–1055. Search this book on
- ↑ Wheeler, Harry E. (1958). "Time Stratigraphy". AAPG Bulletin. 42: 1047–1063.
- ↑ Grabeau, Amadeus W. (1913). Principles of Stratigraphy. New York: A.G Seiler & Co. pp. 726–744 & 821–827. Search this book on
- ↑ Allaby, Ailsa; Allaby, Michael (1999). A Dictionary of Earth Sciences. Oxford University Press. doi:10.1093/acref/9780199211944.001.0001. ISBN 9780191726613. Search this book on
- ↑ Schulz, E.H. (1958). "The Chonosome and the Supersome, terms proposed for low rank chronostratigraphic units". Bulletin of Canadian Petroleum Geology. 30/1: 9–33.
- ↑ Griffiths, Cedric.M.; Nordlund, Ulf (1993). "Chronosomes and Quantitative stratigraphy". Geoinformatics. 4/3: 327–336.
- ↑ Wheeler, Harry E. (1958). "Time Stratigraphy". AAPG Bulletin. 42: 1047–1063.
- ↑ Quayyum, F.C.; Catuneau, O. (2017). "The Wheeler diagram, flattening theory, and time". Journal of Marine and Petroleum Geology. 86: 1417–22851430.
- ↑ Weller, Marvin J. (1964). "Development of the Concept and Interpretation of Cyclic Sedimentation". Kansas Geological Survey Bulletin. 160: 607–621.
- ↑ Kaixuanr, AN; Hanlin, Chen; Xiubin, Lin; et al. (2017). "Major transgression during Late Cretaceous constrained by basin sediments in northern Africa: implication for global rise in sea level". Frontiers of Earth Science. 11 (4): 740–750.
- ↑ Willis, Bailey (1910). "Principles of paleogeography". Science. 31: 241–260.
- ↑ McClure, Norman M.; Brown, Andrew A. (1992). Miller Field, A subtle Upper Jurassic Submarine Fan Trap. 54. South Viking Graben, United Kingdom Sector , North Sea: American Association of Petroleum Geologists Memoir. pp. 307–322. Search this book on
- ↑ Japsen, Peter; Green, Paul F.; Chalmers, James A.; Bonow, Johan M. (2018). "Mountains of southernmost Norway: uplifted Miocene peneplains and re-exposed Mesozoic surfaces". Journal of the Geological Society. 175: 721–741.
- ↑ Pitcher, Wallace S.; Berger, Anthony R. (1972). The Geology of Donegal. London: Wiley-Interscience. p. 132-142. ISBN 0-471-69055-4. Search this book on
- ↑ Goransen, Roy (1938). "Silicate – Water Systems: Phase equilibria in the NaAlSi3O8 – H2O and KALSi3O8 – H2O Systems at High Temperatures and Pressures". American Journal of Science. 35A: 71–91.
- ↑ Atherton, Michael (1983). "Granite magmatism". Journal of the Geological Society. 150: 1009-1023.
- ↑ Thompson, Alan Bruce (1999). Some time-space relationships for crustal melting and granitic intrusion at various depths. Geological Society, London, Special Publications. 168. pp. 7–25. Search this book on
- ↑ Pasyanos, Michael; Walter, William (2002). "Crust and upper-mantle structure of North Africa, Europe and the Middle East from inversion of surface waves". Geophysical Journal International. 149: 463–481.
- ↑ Bachman, O.; Berganz, G.W. (2008). "Rhyolites and their Source Mushes across Tectonic Settings". Journal of Petrology. 49: 2277–2285.
- ↑ Levorsen, Arville I. (1967). Geology of Petroleum. W.H. Freeman and Company. pp. 510–514. Search this book on
- ↑ Dott, Robert H.; Reynolds, Merrill J. (1969). Sourcebook of Petroleum Geology. American Association of Petroleum Geologists. pp. 46–69. Search this book on
- ↑ Bowman, M.B.J.; McClure, N.M.; Wilkinson, D.W. (1993). Wytch Farm oilfield: deterministic reservoir description of the Triassic Sherwood Sandstone. Petroleum Geology of Northwest Europe: Proceedings of the 4th Conference. The Geological Society, London. pp. 1513–1517. Search this book on
- ↑ Dreyer, Tom (1993). Geometry and facies of large-scale flow units in fluvial-dominated fan-delta-front sequences. Advances in Reservoir Geology, Geological Society Special Publication No. 69, London. pp. 135–174. Search this book on
- ↑ Miall, Andrew (2010). The Geology of Stratigraphic Sequences. Berlin, London: Springer-Verlag. pp. 363–379. Search this book on
- ↑ Parkinson, N.; Summerhayes, Colin (1985). "Synchronous global sequence boundaries". Bulletin of the American Association of Petroleum Geologists. 69: 685–687.
- ↑ Dott, Robert H. Jr. (1992). Eustasy. 160. Geological Society of America. Search this book on
This article "Sedimentary isostasy" is from Wikipedia. The list of its authors can be seen in its historical and/or the page Edithistory:Sedimentary isostasy. Articles copied from Draft Namespace on Wikipedia could be seen on the Draft Namespace of Wikipedia and not main one.